Thermodynamics
- Thermodynamics deals with the concepts of heat and temperature and the inter-conversion of heat and other forms of energy.
- The four laws of thermodynamics govern the behaviour of these quantities and provide a quantitative description. William Thomson, in 1749, coined the term thermodynamics.
Laws of Thermodynamics
- Thermodynamics deals with the concepts of heat and temperature and the inter-conversion of heat and other forms of energy.
- The four laws of thermodynamics govern the behaviour of these quantities and provide a quantitative description. William Thomson, in 1749, coined the term thermodynamics.
Zeroth Law of Thermodynamics
- The Zeroth law of thermodynamics states that if two bodies are individually in equilibrium with a separate third body, then the first two bodies are also in thermal equilibrium with each other.
- This means that if system A is in thermal equilibrium with system C and system B is also in equilibrium with system C, then system A and B are also in thermal equilibrium.
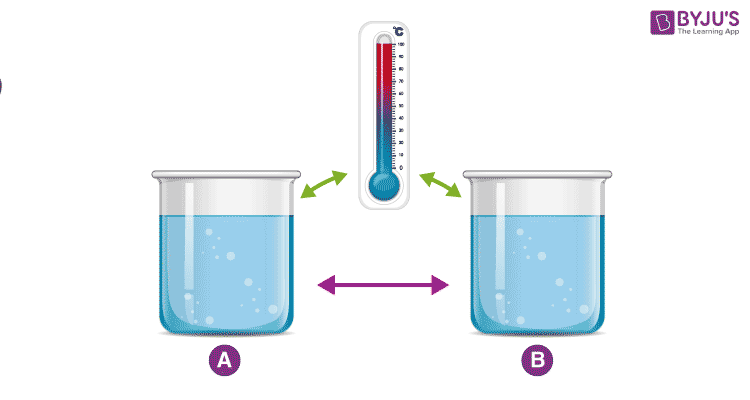
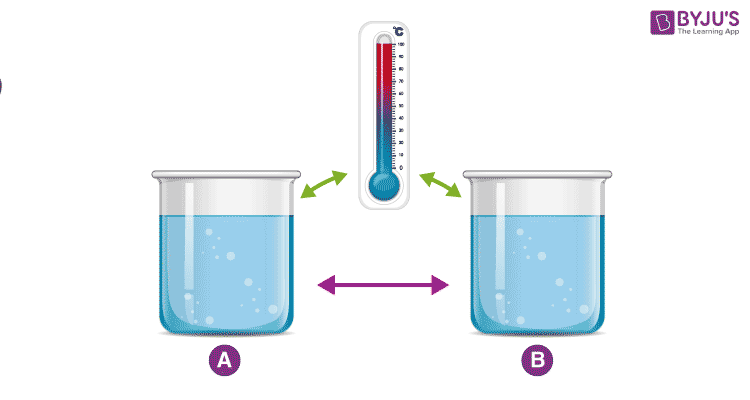
First Law of Thermodynamics
- Relation between heat and work and the concept of internal energy.
- Energy is always conserved, i.e., it can neither be created nor destroyed, but it can be transformed from one form to another.
- Internal energy is a thermodynamic property of the system that refers to the energy associated with the molecules of the system, which includes kinetic energy and potential energy.
First Law of Thermodynamics in Biological Systems
All biological organisms require energy to survive. In a closed system, such as the universe, this energy is not consumed but transformed from one form to another.
Cells, for example, perform a number of important processes. These processes require energy.
In photosynthesis, the energy is supplied by the sun. Light energy is absorbed by cells in plant leaves and converted to chemical energy. The chemical energy is stored in the form of glucose, which is used to form complex carbohydrates necessary to build plant mass.
The energy stored in glucose can also be released through cellular respiration. This process allows plant and animal organisms to access the energy stored in carbohydrates, lipids, and other macromolecules through the production of ATP. This energy is needed to perform cell functions such as DNA replication, mitosis, meiosis, cell movement, endocytosis, exocytosis, and apoptosis.
Why “∆G = ∆H – T∆S” is the most important equation in biochemistry?
- Biochemistry is the study of biologically relevant chemical reactions, mainly those involving carbon-containing molecules.
Cells use the free energy released by spontaneous chemical reactions to drive non-spontaneous chemical reactions, which require an input of free energy. If cells could not "couple" reactions in this way, life itself would not be possible.∆G = ∆H – T∆S
To understand this extremely abstract equation, let’s break it down. Anytime there is a delta (∆), that means “change in.” For instance, if we began with seven cookies and now only have two left, the change in (∆) cookies is -5. The reason we measure change (∆) is because the underlying value might be difficult if not impossible to measure. With the exception of temperature (T), none of the other terms can be measured directly. We can only measure changes.
Free energy (G) refers to energy that is available to do useful work. When you run your laptop or smartphone, the battery is not able to do useful work with all of the stored energy. How do we know? Because those electronic devices get hot. That’s not supposed to happen in an ideal world; it’s wasted energy. But there is nothing we can do about it because we don’t live in an ideal world. (More on that in a moment.) As a result, the change in free energy (∆G) is always less than the total amount of energy that could have been extracted for useful work.
Enthalpy (H) is a slightly odd concept that chemists invented to help them understand energy changes during chemical reactions. The change in enthalpy (∆H) is essentially the change in heat. (It is slightly more complicated than that, as it also includes pressure-volume work. Thankfully, that is often negligible and can be ignored.) Chemical reactions either absorb heat (and become colder to the touch) or release heat (and become warmer to the touch), and the change in enthalpy (∆H) measures this.
T∆S, the final term, represents the product of temperature (T) and the change in entropy (∆S). Remember that ideal world of perfect batteries that we don’t live in? The second law of thermodynamics tells us that entropy (S, which can be thought of as “waste energy” or “disorder” or “chaos”) always increases in the Universe. You can think of entropy as a sort of “tax” that the Universe charges for every energy transfer. T∆S, which represents the quantity of this “tax,” must be subtracted from ∆H.
Let’s try again to restate the equation ∆G = ∆H – T∆S in plain English: The change in the amount of energy available to do useful work (∆G) equals the change in heat (a form of energy transfer) of a chemical reaction (∆H) minus some ridiculous “tax” that the Universe charges (T∆S).
∆G determines whether a chemical reaction can occur
Great. What does that have to do with life? So glad you asked!
Life is complex, and it requires many chemical reactions that do not occur “spontaneously.” A spontaneous reaction occurs with no intervention. If left to its own devices (that is, there is no input of energy), the chemical reaction will occur by itself. Note that this has nothing to do with speed. A spontaneous reaction can be very fast or incredibly slow. Corrosion due to rust formation is spontaneous, but it takes a long time.
For the cells of your body to survive, they need to perform both spontaneous and non-spontaneous reactions. (An example of a non-spontaneous reaction is building a protein.) The way that a cell can perform non-spontaneous reactions is by “coupling” a spontaneous reaction (which is energetically favorable and releases free energy) to a non-spontaneous reaction (which is energetically unfavorable and requires an input of free energy). As long as the overall process results in a net release of free energy (by convention, we consider this a negative ∆G value), the reaction can proceed.
The above figure shows a very typical series of chemical reactions in a cell. A cell can extract free energy from glucose (-∆G) to produce an intermediate and highly energetic molecule (called ATP) that drives protein synthesis, which requires an input of free energy (+∆G). Because the overall process has a net release of free energy (-∆G), the reaction can proceed.
If our cells did not have the ability to drive non-spontaneous, energetically unfavorable chemical reactions (+∆G) using spontaneous, energetically favorable chemical reactions (–∆G), life could not exist. That is why ∆G = ∆H – T∆S is the most important equation in biochemistry.
In simple terms, the endothermic reactions absorb energy from the surrounding that is in the form of heat. On the other hand, an exothermic reaction releases energy into the surrounding of the system.
A popular example of an endothermic chemical reaction is photosynthesis. During this process, plants absorb energy from the Sun and convert it into carbon dioxide and water into glucose and oxygen. An example of an exothermic reaction is the chemical reaction between sodium and chlorine, producing a bright yellow light and a great amount of heat energy.
Endothermic Reactions
The endothermic process is a term that describes a reaction where the system absorbs the energy from its surrounding in the form of heat. A few examples of the endothermic process are photosynthesis, evaporating liquids, melting ice, dry ice, alkane cracking, thermal decomposition, ammonium chloride in water and much more.
As the name implies, ‘endo’ means ‘to absorb,’ and ‘thermic’ means ‘heat.’
This energy is produced as a result of the reaction of reactants into the product. It occurs as a result of the dissociation of the bonds between the molecules. The energy is then released through the formation of new bonds.
Heat is taken up from the surroundings in such reactions, so the temperature of the system where the reaction is taking place remains cooler. Also, at the end of the reaction, the enthalpy, which is the change in heat energy during the conversion of reactants to products, increases.
Exothermic Reactions
The exothermic reaction is the opposite of an endothermic reaction. It releases energy by light or heat to its surrounding. A few examples are neutralisation, burning a substance, reactions of fuels, deposition of dry ice, respiration, solution of sulphuric acid into water and much more.
The term ‘Exo’ refers to ‘to release,’ and ‘thermic’ refers to ‘heat.’
The energy released is caused by the formation of new bonds (products) at a higher level. While the energy required to break up the bonds (reactants) is lower. At the end of the reaction, the enthalpy change decreases as well. During chemical reactions, a great deal of energy is required. This energy was used to maintain the bond that held the molecules together. As a result of the reactions between molecules and compounds, as well as the breaking of bonds, a tremendous amount of energy is released.
Difference between Endothermic and Exothermic Reactions
Q1
In a chemical reaction, why is heat released or absorbed?
Chemical bonds are either broken or formed in any chemical reaction.
Heat is released when chemical bonds form, and heat is absorbed when chemical bonds break.
Since molecules want to remain together, forming chemical bonds between them requires less energy than breaking them, which requires more energy and results in heat being absorbed from the surroundings.
Q2
Chemical bonds are either broken or formed in any chemical reaction.
Heat is released when chemical bonds form, and heat is absorbed when chemical bonds break.
Since molecules want to remain together, forming chemical bonds between them requires less energy than breaking them, which requires more energy and results in heat being absorbed from the surroundings.
Define the enthalpy of a reaction.
The enthalpy of a reaction is defined as the heat energy change (HH, H) that occurs when reactants become products. ΔH is positive if heat is absorbed during the reaction; if heat is released, ΔH is negative.
Q3
The enthalpy of a reaction is defined as the heat energy change (HH, H) that occurs when reactants become products. ΔH is positive if heat is absorbed during the reaction; if heat is released, ΔH is negative.
During the endothermic reaction, what type of energy conversion will take place?
This is the conversion of kinetic energy to chemical energy. Heat is absorbed and converted into chemical energy during an endothermic reaction
Q4
This is the conversion of kinetic energy to chemical energy. Heat is absorbed and converted into chemical energy during an endothermic reaction
Why is respiration regarded as an exothermic reaction?
Since energy is released during the process of respiration, it is an exothermic process. The carbon dioxide in the food is broken down to form glucose during the process. This glucose combines with oxygen in our cells, releasing a large amount of energy.
Q5
Since energy is released during the process of respiration, it is an exothermic process. The carbon dioxide in the food is broken down to form glucose during the process. This glucose combines with oxygen in our cells, releasing a large amount of energy.
What is the main difference between an exothermic and an endothermic process?
The main difference between exothermic and endothermic reactions is that an endothermic reaction absorbs energy in the form of heat from its surroundings, whereas an exothermic reaction releases energy to the surroundings.
The main difference between exothermic and endothermic reactions is that an endothermic reaction absorbs energy in the form of heat from its surroundings, whereas an exothermic reaction releases energy to the surroundings.
Standard enthalpy of formation: CLICK HERE
Thermochemical Equations: CLICK HERE
- The first law of thermodynamics states that the energy of the universe remains constant; though energy can be exchanged between system and surroundings, it can’t be created or destroyed.
- The second law clearly explains that it is impossible to convert heat energy to mechanical energy with 100 per cent efficiency.
- For example, if we look at the piston in an engine, the gas is heated to increase its pressure and drive the piston.
- However, even as the piston moves, there is always some leftover heat in the gas that cannot be used for carrying out any other work.
- Heat is wasted, and it has to be discarded. In this case, it is done by transferring it to a heat sink or in the case of a car engine, waste heat is discarded by exhausting the used fuel and air mixture to the atmosphere.
- Additionally, heat generated from friction that is generally unusable should also be removed from the system.
The Second Law of Thermodynamics Equation
- Mathematically, the second law of thermodynamics is represented as
ΔSuniv > 0
Where ΔSuniv is the change in the entropy of the universe.
- Entropy is a measure of the randomness of the system, or it is the measure of energy or chaos within an isolated system. It can be considered a quantitative index that describes the quality of energy.
No comments:
Post a Comment